Berkeley Lab researchers uncovered a distinctive adaptation that some bacteria use to quickly form protective communities called biofilms, which help them survive in adverse environments. The work could lead to better stewardship of areas with high levels of heavy metals, nutrients, or other forms of hazardous waste.
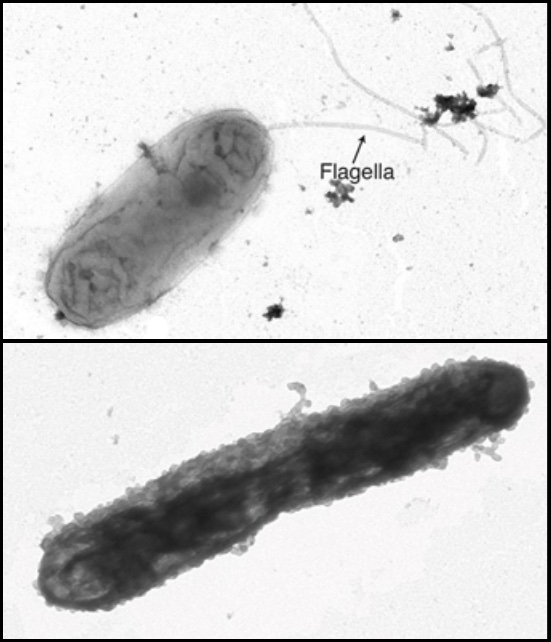
Pollution in surface water and groundwater is a growing global issue, disrupting natural systems and impacting both wildlife and human health. Understanding how different microorganisms function to cycle nutrients through the environment and maintain healthy ecosystems is a key aspect of addressing these challenges. Notably, the bacterial species Rhodanobacter has a documented resilience against high levels of heavy metals and acidity, and plays a particularly crucial role in removing excessive nitrate from groundwater.
A team of ENIGMA researchers led by Mingfei Chen, a postdoctoral scholar in Ecology Department Head Romy Chakraborty’s group, was interested in how Rhodanobacter adapt to these stressors, with the notion that a deeper understanding of their coping mechanisms could refine strategies for managing contaminated areas.
One tactic that Rhodanobacter and other bacteria use to withstand highly polluted environments is through the formation of biofilms: slimy, glue-like substances that help bacteria adhere to surfaces. The distinct structure of these biofilms, which are made up of layers of associated microbial cells surrounded by a protective matrix, shields the microbes from potentially harmful substances in the environment.
In their recent study, Chakraborty’s team, which is based in the Earth and Environmental Sciences Area (EESA), investigated growth and stress response of biofilms formed by different Rhodanobacter strains across varied nutrient, pH, nitrate, and metal conditions. As expected, increased levels of nutrients, acidity, and heavy metals enhanced biofilm formation across the board. But the researchers did observe an adaptive strategy they did not anticipate: While most bacterial species require flagella—tiny tail-like structures used for movement—to form a biofilm, Rhodanobacter loses their flagella when exposed to high levels of aluminum, even as biofilm growth increases. The finding not only underscores Rhodanobacter‘s specialized resilience in these extreme environments, but also provides a fresh insight as to how biofilms form.
In addition, the researchers found that genetically similar Rhodanobacter strains behaved analogously even in different environments, which helped them to identify key genes that allow these bacteria to persist under stress.
This enhanced genetic understanding, taken in conjunction with the findings about aluminum stress driving biofilm growth and flagella loss, paint a clearer picture of how Rhodanobacter survive in extreme conditions. The researchers anticipate that these findings could extend beyond Rhodanobacter to provide a broader understanding of microbial adaptation, and potentially serve as the basis for managing bacteria in contaminated environments.
Additional Biosciences contributors for this work include:
Environmental Genomics and Systems Biology research scientist Valentine Trotter, senior scientist Adam Deutschbauer, senior faculty scientist Adam Arkin, research scientist Lauren Lui, and research associate Andrew Hendrickson; Molecular Biophysics and Integrated Bioimaging retiree affiliate Peter Walian; and Biological Systems and Engineering staff scientist Chris Petzold and research scientist Yan Chen.