One major source of treatment failure for cancer patients is tumor resistance to chemotherapy with alkylating agents. This class of commonly prescribed drugs is thought to curb the rapid replication of cancer cells by damaging DNA. The prevailing narrative of how chemotherapy resistance arises has historically focused on the suite of molecules that respond after DNA damage occurs. But an emerging body of evidence suggests that some of these cleanup mechanisms interface with RNA as well, which could challenge the current understanding of how cancer cells and chemotherapy drugs interact.
“It’s not a clean story,” said Susan Tsutakawa, a biochemist staff scientist and Head of the Department of Structural Biology Department in the Molecular Biophysics and Integrated Bioimaging (MBIB) Division. “It’s a lot of breadcrumbs.”
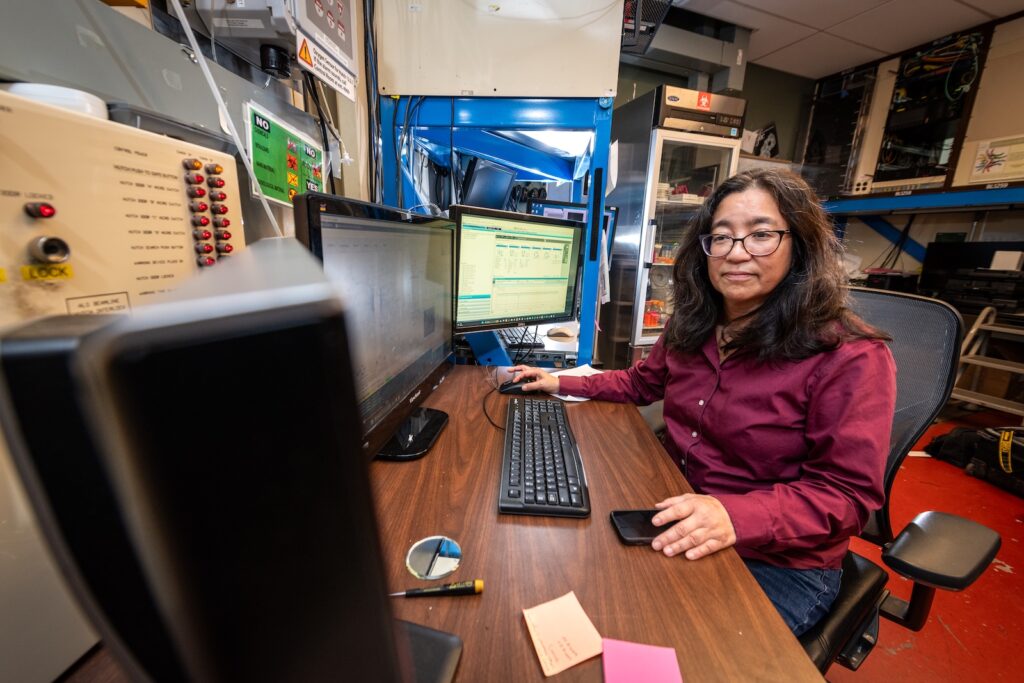
Tsutakawa and a team of researchers with the Structural Cell Biology of DNA Repair Machines (SBDR) Program have followed the breadcrumbs to Activating Signal Cointegrator Complex subunit 1 (ASCC1), the least-studied protein in the family of enzymes that respond to DNA damage. Results from their recent study, which include the first-ever structural analysis of ASCC1, implicate ASCC1 as a biomarker that may predict a patient’s response to chemotherapy. Their findings also support the notion that RNA-mediated processes may play a larger role in cancer treatment outcomes than previously thought.
Certain cancer cells appear to have a special relationship to the family of damage-response that ASCC1 is a part of: they pump them out more generously than normal cells and are more susceptible to the effects of alkylating chemotherapy when there’s less of them to go around.
“While these proteins may be doing something in normal cells, normal cells may not be dependent on them.” Tsutakawa said. This could mark a vulnerability, highlighting a promising path towards drugs that target cancer cells while leaving normal cells relatively unscathed.
Armed with the knowledge that these proteins clearly affect some aspects of cancer pathology, the team consulted population-scale genetic data made publicly available through The Cancer Genome Atlas (TCGA) project on ASCC1. They discovered that a high level of ASCC1 expression was associated with increased mutations and poor patient survival in several tumor types. The effect was particularly pronounced for pancreatic cancer patients. These findings suggest that high ASCC1 expression could serve as a red flag for genetic instability and poor prognosis for some cancers, and underscore ASCC1’s importance in understanding cancer therapy response.
Leveraging specialized imaging capabilities at Berkeley Lab’s Advanced Light Source (ALS), Tsutakawa’s team then zoomed in on ASCC1 at a molecular scale in the hopes that resolving its structural and biochemical signatures would reveal clues about how it acts to impact cancer treatment outcomes. They used X-ray crystallography to capture a super high-resolution snapshot of ASSC1’s crystalline structure and small-angle X-ray scattering (SAXS)––a solution-based technique that can follow dynamic movements––to gauge how ASCC1 behaves. Together, the two approaches uncovered never-before-seen features of ASCC1 that point towards its biochemical and cellular functions. In particular, structural analysis revealed two areas of the protein capable of recognizing RNA and its associated machinery. Using gel electrophoresis, a technique for separating proteins by charge or size, the researchers then confirmed that a high proportion of ASCC1 bound RNA in solution.
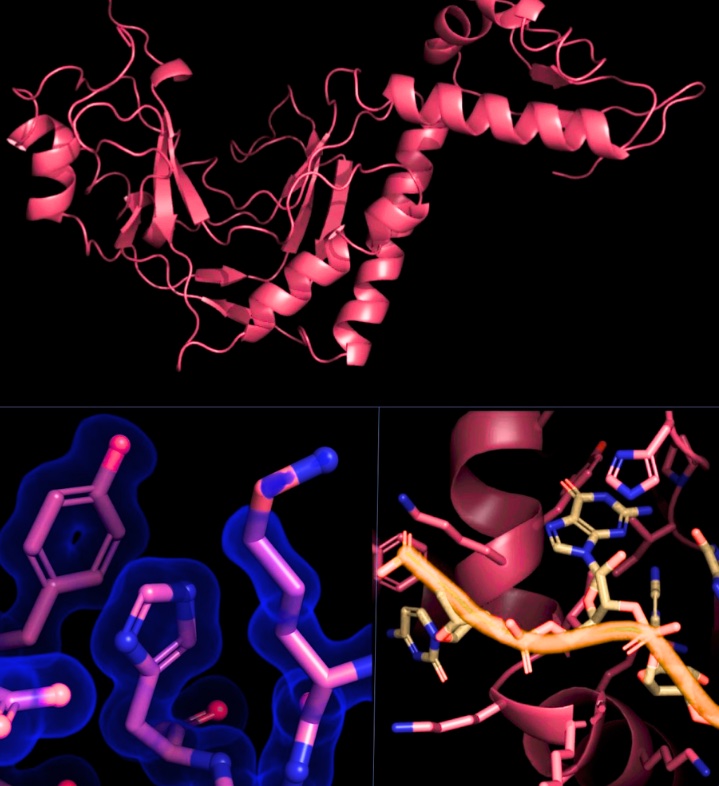
“ASCC1 was not only interacting with RNA; it was specific for RNA,” said Tsutakawa. “That’s building the story that chemotherapy with alkylating agents may impact RNA, not just DNA, which adds nuance to how we think about resistance mechanisms.”
Their findings support a strong case that both RNA and ASCC1 are key players in this puzzle, but there’s still some detective work to do in terms of parsing specific causal chains and mechanisms. As a first step towards using their structural toolkit to explore ASCC1’s possible biological functions in the chemotherapy response pathway, the researchers mapped data on cancer-associated mutations onto the structure of ASCC1 that they had determined. They found that most of the mutations scored to be important mapped to structural elements involved in ASCC1 enzymatic activities or RNA binding, which is consistent with the premise that mutations localized to a functional site are more likely to impact cancer activity.
“When a patient gets a biopsy and has single-gene sequencing done, doctors may find mutations and not know if a mutation is important or not,” Tsutakawa said. “Our scoring system indicates mutations that could have the biggest impact based on where they are on our protein structure.”
These flagged areas could be promising targets for further testing on activity and function, and eventually could inform the development of useful diagnostic and prognostic tools.
Other Biosciences Area contributors to this work include: Biological Systems and Engineering Division biologist research scientists Altaf Sarker and Miaw-Sheue Tsai and MBIB staff scientist Michal Hammel and former graduate research associate Daniel Rosenberg.