Improving Climate Predictions by Unlocking the Secrets of Soil Microbes
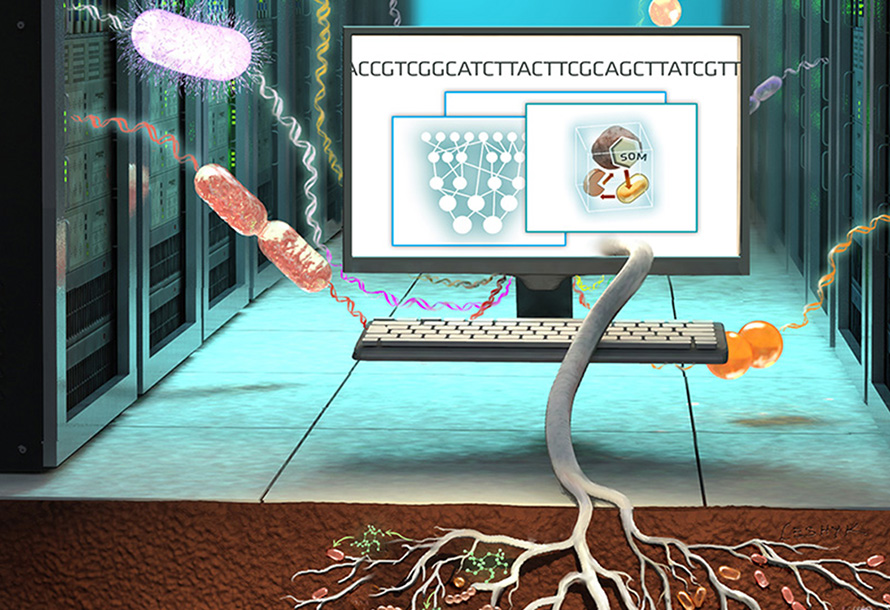
A Berkeley Lab–led team of scientists has developed a new model that incorporates genetic information from microbes, enabling them to ascertain how soil microbes store carbon supplied by plant roots. The model could inform agricultural strategies to preserve carbon in the soil, supporting both plant growth and climate change mitigation.
More »