Biosciences Area researchers have developed a novel approach for studying the FLASH effect, an emerging frontier in radiation oncology.
Radiation therapy is currently the standard of care for more than half of all cancer patients. Despite technological advances that have improved its power and precision, conventional radiotherapy still has its limitations, particularly for radiation-resistant tumors. Cranking up the delivery dose, which is often required to completely eliminate cancerous tissue, can induce serious side effects in healthy surrounding tissue.
Though it may seem counterintuitive, delivering ultrafast, high-intensity doses of radiation to tumors can actually reduce the toxicity to surrounding healthy cells, while still directing a potent anti-cancer effect towards the target. Scientists have documented this perplexing phenomenon—dubbed the FLASH radiotherapy effect—in both cell lines and animal models, but they have yet to confirm how or why it works. A new experimental platform that uses X-rays to investigate the FLASH effect brings science a step closer to clarifying its underlying mechanisms, laying the foundation for major strides in the field of radiation oncology.
“It’s really surprising that higher dose rates can actually protect tissues,” said Corie Ralston, a staff scientist in the Molecular Biophysics and Integrated Bioimaging Division (MBIB) and director of the biological nanostructures facility at the Molecular Foundry. “It’s a giant puzzle.”
A team co-led by Ralston and Antoine Snijders, a senior scientist and Head of the Bioengineering & Biomedical Sciences Department in the Biological Systems and Engineering (BSE) Division, developed the approach. Their framework, which involves two novel components, was published this past November in Radiation Research.
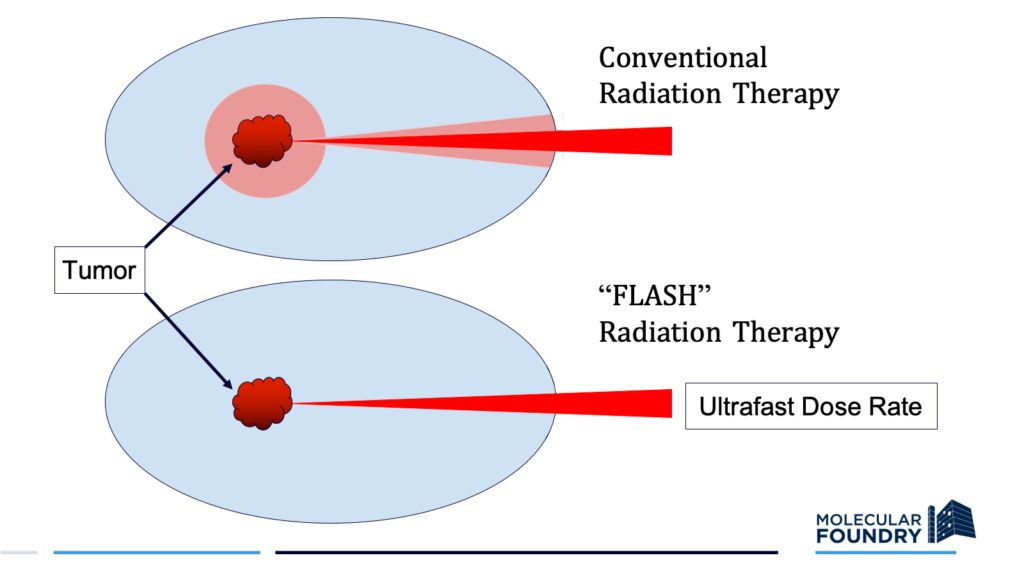
The researchers took advantage of Berkeley Lab’s advanced experimental facilities to directly compare the effect of conventional and FLASH radiotherapy techniques, using a short peptide in water as an experimental model. For the low dose rate, conventional radiation condition, they irradiated peptide samples on Berkeley Lab’s XRAD machine, a self-contained X-ray system for delivering low dose rate radiation; for the ultrafast, FLASH radiation condition, they irradiated samples on the synchrotron beamline 3.3.1 at the Advanced Light Source (ALS).
“No other lab in the world, as far as we know, has both capabilities in one place,” said Ralston.
The researchers then assessed the impacts of these two conditions at varying dose amounts with an unconventional application of X-ray footprinting mass spectrometry (XFMS), a structural biology method which uses X-rays to precisely probe the oxidation patterns and reactivity of proteins in water. While XFMS is typically used to characterize protein structure, the researchers in this case were interested in peptide oxidation as a way to measure how much each radiotherapy treatment induced modifications or damage.
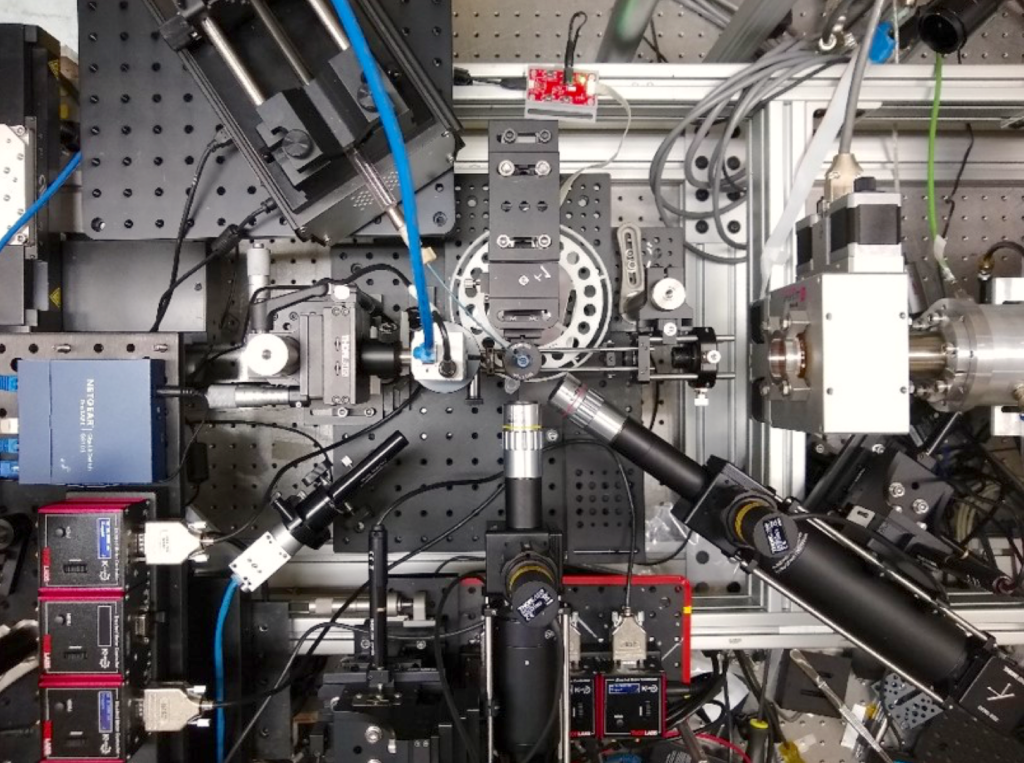
They found that fewer radiation-induced changes occurred in peptides exposed to radiation at ultrafast FLASH rates than those irradiated at conventional rates, a result consistent with previous reports that higher dose rates can result in reduced damage.
“We were able to detect the FLASH effect in this simple system of peptides in water,” Ralston said. The result signals the power of their experimental design for studying the dynamics of FLASH radiotherapy in more depth.
One hotly debated theory for how FLASH radiotherapy allows tissues to tolerate higher doses is that the rapid delivery of radiation induces oxygen depletion, providing a protective effect for normal tissues, but not cancer cells. To investigate this hypothesis, the researchers ran the experiment under both oxygen-rich and oxygen-poor conditions, and observed lower rates of oxidation in the oxygen-starved peptides. Although this does not confirm the precise mechanism of the FLASH effect, it does point to oxygen as a critical player and sets the stage for ongoing exploration of how, exactly, oxygen depletion mediates the peptide damage pathway.
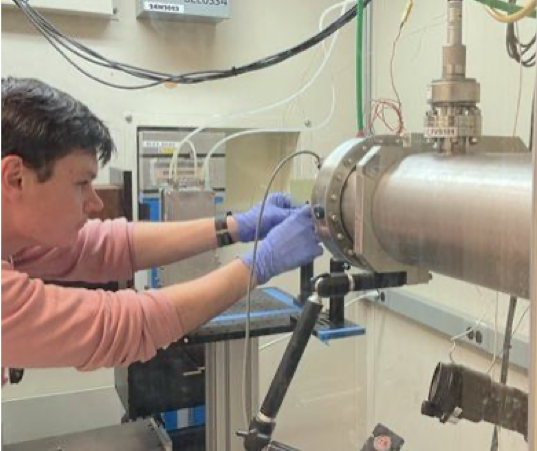
The precision and control of this new methodology will allow scientists to thoroughly quantify the extent of oxidative damage across a wide range of dose parameters for both conventional and FLASH approaches, a vital step in assessing the safety criteria of different radiation treatment regimes. The researchers hope to build on their work in simple peptides to study the FLASH effect first in full proteins, then cell lines, and eventually both healthy and cancerous tissues.
“Fundamental insight into how the FLASH effect works will allow radiation treatments to be better tailored to the type of tumor, and will help make radiation therapy more effective in treating cancer,” said Ralston.
Other Biosciences Area contributors to this work include: MBIB research scientist Sayan Gupta; BSE research scientist Jamie Inman; MBIB faculty scientist Susan Marquesee; and BSE senior scientist Jian-Hua Mao. Researchers from Berkeley Lab’s Accelerator Technology and Applied Physics Division, including research scientists Kei Nakamura and Lotti Obst-Huebl and graduate research assistant Jared de Chant, calibrated dose amounts and rates on the beamline. Collaborators at University of Lausanne, Switzerland, provided a FLASH comparison using electron irradiation to benchmark X-ray experiments. Additional contributors included researchers at University of California, Berkeley.
Read more in the ALS Science Brief.