Pulley systems allow something heavy to be lifted by decreasing the amount of force needed to displace the object. This can be accomplished by distributing the force over a number of ropes or cables. Physical pulley systems, such as those integral to the operation of elevators and garage doors, are used every day. It turns out that microbes have a bioenergetic pathway in constant use that acts like a pulley system—only instead of heavy objects and ropes, it uses proteins, electrons, and energy.
This process, called electron bifurcation, allows microbes to create high energy electrons that can be held in reserve in proteins and then used to make or break bonds in specific reactions, such as those that produce critically needed metabolites. A class of chemical reaction found only in biology, electron bifurcation liberates two electrons from one donor and adds one each to two other spatially separated electron acceptors. Understanding this critical pathway is important for modeling microbes and their metabolisms.
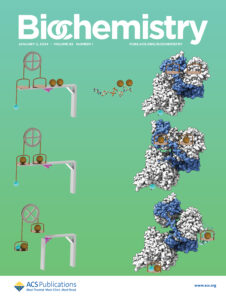
A team of researchers led by Greg Hura, Molecular Biophysics and Integrated Bioimaging Division Deputy for Science, has used synchrotron technology unique to the Advanced Light Source (ALS) at Berkeley Lab to understand a protein involved in this recently discovered microbial bioenergetic pathway. In a Biochemistry article published in November 2023, Hura and colleagues identified motions in the electron bifurcating flavoprotein EtfABCX that help it perform its function and connected the observed motions to its catalytic cycle.
The protein ferredoxin, used in many metabolic pathways that provide energy to organisms, depends on an iron–sulfur cluster integral to its structure to hold high energy electrons. EtfABCX uses electron bifurcation to create the high energy electron so that it can be loaded onto ferredoxin. In this process, two electrons of medium potential get pulled off a metabolite, NADH, which is a type of B vitamin. Like the pulley system, one electron is elevated in energy at the expense of lowering the energy of the second. In this case, the EtfABCX channels the higher energy electrons to the iron cluster in ferredoxin, which can then help the microbe perform high energy bond breaking or making as necessary for microbial metabolism.
One of the tricks EtfABCX employs is to couple electron transfer to conformational change. In experiments performed on the SIBYLS beamline at the ALS, the researchers studied the mechanism of electron channeling in the bifurcation process. Using small angle X-ray scattering (SAXS), a solution-based technique that does not restrain motion, EtfABCX’s steps, triggers, and coordinated conformational change were elucidated. They found that NADH activates the protein’s mechanics, allowing it to act as the wheel and ropes of the pulley system. Once electrons are removed, the protein occupies a new conformation that allows the hand-off of electrons between different regions of EtfABCX.
By following the mechanics and conformations of EtfABCX, the researchers were able to elucidate this novel biological mechanism where energetic electrons are channeled over relatively large distances. Understanding these novel bioenergetic systems will help build microbial energy flux models and aid in the annotation of newly discovered genes.
Other MBIB scientists involved in this study were postdoctoral researcher Daniel Murray, former graduate research associate Daniel Rosenberg, staff scientist Michal Hammel, and former postdoctoral researcher Jan Bierma. The team included collaborators from UC Riverside and the University of Georgia, Athens.
Read more in the ALS Science Brief.