Living organisms possess an unparalleled talent for synthesizing complex molecules with high selectivity, while non-living materials comprise the most efficient energy collection systems. Scientists have sought to unite these complementary strengths by electrochemically coupling separate microbial and inorganic catalysts to form biohybrid systems. Such biohybrid systems are attractive as a sustainable technology to produce fuels and high-value chemicals using renewable energy.
But, a fundamental challenge in designing biohybrid systems is that the environments that support optimal function of living cells and inorganic materials are chemically incompatible, resulting in toxicity, corrosion, or efficiency-degrading cross reactions. To date, the approach has been to keep the biological and abiotic components physically separated by macroscopic (millimeter to centimeter) distances. However, this exacts a high cost in terms of efficiency due to resistance losses (on the order of 25 percent of the cell voltage) caused by ion transport between the components, making scale-up to commercially relevant levels impractical.
Now, Biosciences researchers have developed a novel nanoscale membrane embedded with molecular wires that simultaneously chemically isolates, yet electrochemically couples, a microbial and an inorganic catalyst on the shortest possible length scale. This new modular architecture, described in a paper recently published in Nature Communications, opens up a large design space for building scalable biohybrid electrochemical systems for a variety of applications, including electricity generation, waste remediation, and resource recovery, in addition to chemical synthesis.
The work was led by Heinz Frei, a senior scientist in Molecular Biophysics and Integrated Bioimaging (MBIB), and Caroline Ajo-Franklin, staff scientist with the Molecular Foundry who holds a secondary appointment in MBIB. As a proof-of-concept, the researchers electrochemically coupled Shewanella oneidensis, an anerobic bacterium notable for its ability to reduce heavy metals via cytochromes present on the outer membrane, to an inorganic catalyst, tin dioxide. The 2-nanometer-thick silica membrane contains polymers that have functional cyano and nitro chemical groups that transfer electrons, enabling current flow while blocking oxygen and other small molecule transport.
“This advance introduces a completely new architecture for bioelectrochemical systems based on nanoscale integration, and provides a path forward to scaling up these systems to a commercially relevant level,” said Frei. “What’s more, it provides an example of how a key design principle inspired by biology is applied for solving a major scientific gap of engineered systems.”
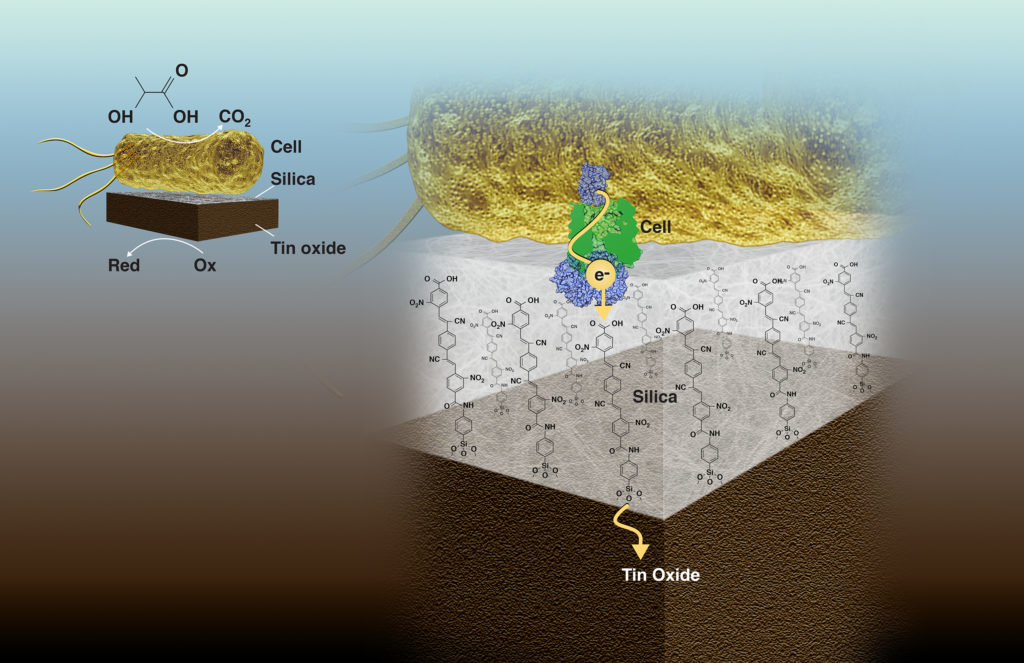
This study builds on previous work by Frei’s group in which they fabricated a square-inch sized artificial photosystem, in the form of an inorganic core-shell nanotube array, and by Ajo-Franklin’s group in which insight at the molecular level revealed how the outer cell membrane protein interacts with an inorganic oxide surface.
The three co-first authors on the new paper were Jose Cornejo, a postdoctoral fellow in Ajo-Franklin’s group, and Hua Sheng and Eran Edri, former postdoctoral fellows in Frei’s group, now at the Chinese Academy of Sciences and Ben-Gurion University, respectively.
This work was supported by the Laboratory Directed Research and Development (LDRD) Program of Berkeley Lab. The Molecular Foundry is a DOE Office of Science User Facility specializing in nanoscale research. A portion of the work was performed at the Joint Center for Artificial Photosynthesis, a DOE Energy Innovation Hub, supported through the DOE’s Office of Science.