X-ray crystallography is a powerful experimental technique for deducing the molecular structure of a material, down to the placement of individual atoms. However, as the name implies, it requires crystals: specifically, purified samples of the molecule of interest, coaxed into a crystal form. And many materials can’t be grown into large, single crystals. Instead they form granular powders made up of a million tiny crystals in random orientations.
Nicholas Sauter, a computer senior scientist in the Molecular Biophysics and Integrated Bioimaging (MBIB) Division, is co-leading a team working to provide a better way for scientists to study the structures of the many materials that don’t form tidy single crystals, such as solar absorbers and metal-organic frameworks: two diverse material groups with huge potential for combating climate change and producing renewable energy.
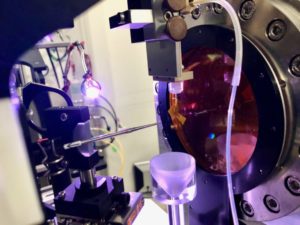
Their new technique, called small-molecule serial femtosecond X-ray crystallography, or smSFX, supercharges traditional crystallography with the addition of custom-built image processing algorithms and an X-ray free electron laser (XFEL). The XFEL, built from a fusion of particle accelerator and laser-based physics, can point X-ray beams that are much more powerful, focused, and speedy than other X-ray sources for crystallography. The entire process, from X-ray pulse to diffraction image, is completed in a few quadrillionths of a second.
Project co-lead and MBIB research scientist Aaron Brewster and MBIB project scientist Daniel Paley developed the algorithms needed to convert XFEL data into high-quality diffraction patterns that can be analyzed to reveal the unit cell – the basic unit of a crystal that is repeated over and over in three dimensions – of each tiny crystalline grain within the sample.
In a paper published in Nature, the team demonstrated proof-of-principle for smSFX and reported the previously unknown structures of two metal-organic materials known as chacogenolates, which have semiconducting and light-interaction properties.
Read the press release in the Berkeley Lab News Center and/or the Computing Sciences news feature.